Electric trucks (eTrucks) are entering markets worldwide. As these vehicles are deployed, fleet operators must determine potential use cases, such as last-mile delivery, and select the best charging strategies. We have identified the use cases most likely to electrify first and the most viable charging strategies based on cost, practicality, and other factors.
Charge of the eTruck brigade
McKinsey estimates eTruck adoption will exceed 30 percent by 2030 across different vehicle classes: light commercial vehicle (LCV), medium-duty truck (MDT), and heavy-duty truck (HDT). The potential uptick in adoption will likely be a result of the alignment of several key factors: regulation, electric truck supply, improvements in battery electric technology, and overall economics.
First, the regulatory push to reduce emissions is driving interest in eTrucks across the logistics and transport sectors, which currently contribute 3 to 5 percent of global CO2 emissions. Regional regulations, such as those in the European Union, require new trucks to reduce CO2 emissions by 30 percent by 2030. States and cities are creating regulatory push as well. For example, California’s recent Advanced Clean Truck regulation requires manufacturers of commercial vehicles to start selling eTrucks in 2024 and restrict all sales to this category by 2045. Likewise, more than 40 cities around the world have begun to ban diesel and gasoline internal combustion engine (ICE) trucks in their city centers.
Next up is supply. While most people would have difficulty naming an eTruck model today, this is already changing. Based on our analysis of automaker future product plans, we expect a five- to tenfold increase in the supply of eTrucks by 2030. Major OEMs have already announced new models across different weight classes, and we expect there will be more than 30 models by 2040.
Technologic and economic factors will also play a big role in eTruck uptake. Improvements in battery and electric powertrain technology, including those related to safety, energy density, battery supply, and performance, are increasing both vehicle range and consumer confidence. As the technology improves, battery costs are also falling. Over the next decade, they should decline to the point where the total cost of ownership (TCO) for many eTruck models will be similar to or better than the TCO for ICE trucks.
These factors will create a favorable environment for the adoption of eTrucks across the LCV, MDT, and HDT segments. Our analysis of three key markets—China, Europe, and the United States—suggests that demand could reach 2.7 million units by 2025 and 11 million units by 2030.
Potential use cases
Our research revealed that eTrucks could play a role across multiple use cases, although these will vary by vehicle class and application (Exhibit 1). Each use case will differ in terms of required range, payload, route predictability, infrastructure access, model availability, and the need for a cold chain (an uninterrupted series of refrigerated production, storage, and distribution activities for goods). Today’s battery packs can best support use cases with predictable routes, relatively shorter ranges, and payloads that do not require cold chains. Thus, we expect three commercial use cases to gain traction first: last-mile delivery, dry goods distribution, and point-to-point long-haul transport.
Use case 1: Light commercial vehicles making last-mile deliveries
This first use case will involve LCVs making last-mile deliveries. These routes are typically short—under 100 kilometers per day—and do not involve heavy or refrigerated payloads. Today’s batteries can easily move these small lightweight trucks, even when they are loaded with packages. In addition, the batteries in these vehicles can easily support these routes, often without the need for en route charging. For instance, a 100 kilowatt-hour (kWh) battery can easily support an LCV driving approximately 130 kilometers per day.
Use case 2: Medium-duty vehicles distributing dry goods
In this use case, MDTs drive across cities or states for dry goods distribution, which typically means delivering goods from warehouses to urban supermarkets or distribution centers. This use case is feasible over the short term because the eTrucks have a defined and fixed daily route and a known average daily distance traveled of about 200 kilometers. Since their fixed routes have known stop times at either end, the eTrucks can be charged at the beginning and end of their journeys.
Use case 3: Heavy-duty vehicles doing point-to-point long haul
This use case covers large heavy trucks—class 7 and class 8—that drive long distances to deliver goods from ports or production facilities to large distribution centers or warehouses. The exact routes and distances remain highly predictable and fixed, with a range of 400 kilometers or more. These eTrucks typically operate almost continuously using two drivers. Given the extreme service cycles, these use cases will likely emerge last and will probably require en route charging at predetermined stops.
Would you like to learn more about the McKinsey Center for Future Mobility?
Potential charging strategies
While eTrucks have yet to be adopted at scale, we have identified five potential charging options that vary by location, cost, feasibility, and flexibility (Exhibit 2). For instance, some strategies call for eTrucks to be charged at hubs where vehicles park while others involve warehouses where they load and unload, public charging stations, or rest stops that feature en route chargers. The initial costs for each charging solution will include charger hardware, installation, any storage or distributed generation equipment, one-time site construction and grid connection costs, or one-time software-development fees. Ongoing operating costs will include maintenance, electricity, demand charges, and labor.
For strategies that use wired charging, two technologies are available:
- alternating-current (AC) charging, also called Level 2 charging, which is relatively slow and has power under 22 kilowatts
- direct-current fast charging (DCFC), which has power over 50 kilowatts
Level 2 chargers are considerably more affordable, ranging in cost from $2,500 to $5,000, while DCFC chargers, ranging from 50 kilowatts to 100 kilowatts, cost anywhere from $15,000 to more than $40,000.
Of the five strategies, we believe that overnight-only charging and overnight and midroute charging are most feasible. These options have predictable and manageable up-front capital expenditures and operating costs, use established technologies, and offer flexibility. The other choices will remain niche applications or are commercially impractical.
Overnight-only charging
Following this strategy, operators charge their fleets overnight at the hub or depot where they park. This approach assumes that eTrucks run for only one shift a day, are idle throughout the night, and usually have a battery that is large enough to support the daily required range when fully charged. Overnight-only charging is possible with today’s technology and infrastructure and could be the most cost-effective charging solution.
Fleets can use less expensive, slower Level 2 chargers at the hub or depot, given the long rest cycles, which limits their up-front capital expenditures; additionally, with overnight charging trucks would charge at night when electricity is cheaper. Alternatively, the fleet operator could use a third-party charging infrastructure provider and lease the charging equipment—a strategy that would avoid capital-expenditure outlays but likely involve slightly higher overall prices.
Overnight and midroute charging
With this option, operators charge eTrucks overnight at the depot and over the day at fast chargers. Midroute chargers can be located at private rest stops, destination warehouses, public retail locations, public charging stations, or truck rest stops. An overnight-and-midroute-charging strategy makes sense when the battery pack is not large enough to support the entire daily required range, even when fully charged.
This charging solution reduces the required capex investments at the depot for both fleet operators and charge-point operators, compared with overnight only, because operators can have smaller battery packs in their vehicles and therefore need fewer depot chargers.
The main considerations for this strategy involve the additional cost for midroute infrastructure build-out or use and the need to guarantee availability. If operators decide to build dedicated midroute stops, capital expenditure will increase. Similarly, operators that use public charging stations midroute will pay more per kilowatt-hour consumed than they do at hubs. For fleets that opt to use public networks, charging stations must be available and operational when needed or else on-time performance might suffer.
Midroute-only charging
If fleet operators select a midroute-only charging method, they will rely exclusively on public or private charging midroute. This model reduces the required infrastructure investment, but it is only feasible in locations where public charging stations exist along the desired route or if electricity costs are low enough to justify a switch to exclusive midroute charging. As any charging strategy, this option has several drawbacks. For instance, a fleet’s overall idle time could increase if chargers are unavailable.
Midroute-only charging is most suitable for fleet operators with little or no depot parking space, such as car-on-demand operators with free-floating fleets, as well as limited daily mileage or long breaks between cycles. Even when such enablers are in place, we expect that fleet operators will need to enter targeted arrangements with charge-point operators to ensure charging access and avoid interference with fleet performance.
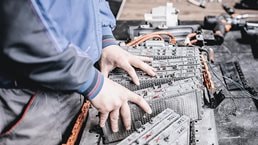
Improving battery-electric-vehicle profitability through reduced structural costs
Battery swapping
In this strategy, eTruck operators can remove discharged batteries and replace them with fully charged ones, either at the hub or depot location or midroute at dedicated swapping stations. The main advantage with this charging strategy is time. Swapping stations will have a stock of already-charged batteries that can be installed in much less time than it would take for a wired charger to charge an onboard battery.
While faster, the costs associated with this model could be considerable. Operators will need to set up a charging and storage hub for the battery packs, stock more than one battery per truck, and potentially hire labor to conduct the swapping. If a fleet operator has two different truck types with different battery-pack configurations, costs could escalate even further. That said, there are some use cases where battery swapping makes sense, such as those involving travel through remote areas that lack public-charging infrastructure. Battery swapping may also be the best solution when turnaround times for charging are critical or when a fleet operator only uses one truck brand. No operators have yet deployed battery swapping on a commercial scale.
Overhead catenary charging
In this solution, the eTruck itself has a small battery or lacks one. It drives on a fixed route while drawing power from an overhead catenary—a line or wire that directly provides electricity to vehicles. This strategy allows the fleet operator to avoid high expenses related to eTruck batteries. Savings can be considerable, since battery expenditures usually represent about 40 to 60 percent of the overall capital expenditures for these vehicles.
A major disadvantage of catenary charging is the extensive capital expenditures required to build the overhead power grid, as well as the need for permanent fixed routes. Since only a few routes are now equipped with this technology, this solution is not commercially feasible in most instances. Beyond areas where the infrastructure already exists, catenary charging might make sense for very short fixed transfers with heavy payloads, such as in mining applications.
Considerations when choosing a charging strategy
To test the viability of each strategy, we focused on the use cases that are most feasible over the short term: last-mile delivery, dry goods distribution, and point-to-point long-haul distribution. For each case, we then performed a TCO analysis for three different charging options: overnight only, overnight plus midroute with the same battery size, and overnight plus midroute with a smaller battery size. In addition to considering the capital expenditure for the battery pack and charging infrastructure, we looked at all operating expenditures for retail electricity and the charging infrastructure—both operations and maintenance (Exhibit 3). We also factored in costs that would be the same across different charging solutions, such as those for vehicle chassis, electronics capital expenditures, and vehicle-maintenance operating expenditures. Our findings highlighted four important points:
- Overnight-only charging is the cheapest choice. For every type of eTruck and route, overnight-only charging is by far the cheapest possible option, largely because energy costs overnight at a hub are lower than those associated with strategies that involve midroute charging. Additionally, operators benefit from using slower, less expensive chargers at hubs. For use cases where eTrucks run more than a single shift or need faster charging at the hubs, the cost differential will be lower. Overnight-only charging is expected to be the dominant solution, at least in the near term, since the technology is already available and most battery packs are expected to be large enough to meet daily route needs on a single charge.
-
The real benefit of midroute charging involves the trade-off between charging and battery costs. Midroute charging serves two purposes. First, it allows eTrucks to drive longer routes than they could serve on a single charge. Second, it could allow eTrucks to use a smaller battery while maintaining the desired range.
Public charging will always be more expensive than depot charging because charge-point operators will seek premium fees on their electricity or service to recoup their infrastructure costs and make a profit. If battery prices for eTrucks remain close to today’s average of $250 to $300 per kilowatt hour, it makes sense to reduce battery capacity using midroute charging, even when electricity prices are very high (over the current average of $0.5 per kilowatt hour for ultra-fast charging). If battery prices decline as expected, public charge-point operators must reduce their fees at fast-charging points below $0.4 per kilowatt hour to remain competitive. Otherwise, fleet operators will find it more cost-effective to opt for bigger batteries and overnight-only charging solutions.
-
Charging technology depends on vehicle size and use. LCVs and MDTs used in single-shift, overnight-only situations can charge with slower Level 2 chargers, even if they have larger batteries. The rating for the standard Level 2 charger is 22 kilowatts. At that power rating, the charger can supply approximately 220 kWh1 of electricity over ten hours.
In the near term, routes for LCVs and MDTs will probably not exceed 200 kilometers, which means they will use less than 220 kWh of energy per day. However, eTrucks driving more than 200 to 300 kilometers per day will require more than 220 kWh and will need faster chargers at the hub. Heavy-duty, long-haul vehicles will always require faster DCFC charging given their routes of more than 400 kilometers and their much larger battery packs (over 300 kWh). If these vehicles used a Level 2 charger, the charging process would take over ten hours, making it even inappropriate for overnight solutions.
-
The business model matters just as much as the technology. While an operator’s selected charging strategy is important, the chosen business model could have even greater implications and be a greater source of innovation. When preparing to launch eTrucks, fleet operators will be more responsive to a seamless model that integrates the design, deployment, and operation of the charging infrastructure with the purchase of the vehicle.
For fleet operators to deploy the charging infrastructure independently, they would have to understand requirements for all elements, including hardware, installation, and software. They would then need to scan the market, evaluate multiple vendors, and engage one. That may change, however, since integrated solutions are starting to emerge in which OEMs or independent providers offer a “charging as a service” model that provides a one-stop-shop option in which a single provider covers everything—solution design, procurement, installation of hardware, operations, and maintenance. There are also new economic models that support these types of solutions, such as a dollar-per-mile or dollar-per-kWh fully loaded cost. Such innovations help eliminate high up-front costs and provide higher levels of predictability over the long term.
As automotive players prepare to launch a range of new eTrucks in markets around the world, the industry must simultaneously solve its charging problems. To encourage strong momentum for eTrucks, the extended ecosystem of OEMs, suppliers, fleets, service providers, and municipalities must collaborate with regulators to ensure charging infrastructure development keeps up with eTruck demand. Without this building block in place, even the most important use cases may see little uptake.